Can gene-editing help solve the energy crisis?
Published on 6th Apr 2021
What role can biofuels play in fighting climate change and can CRISPR sequencing technology help it reach its potential?
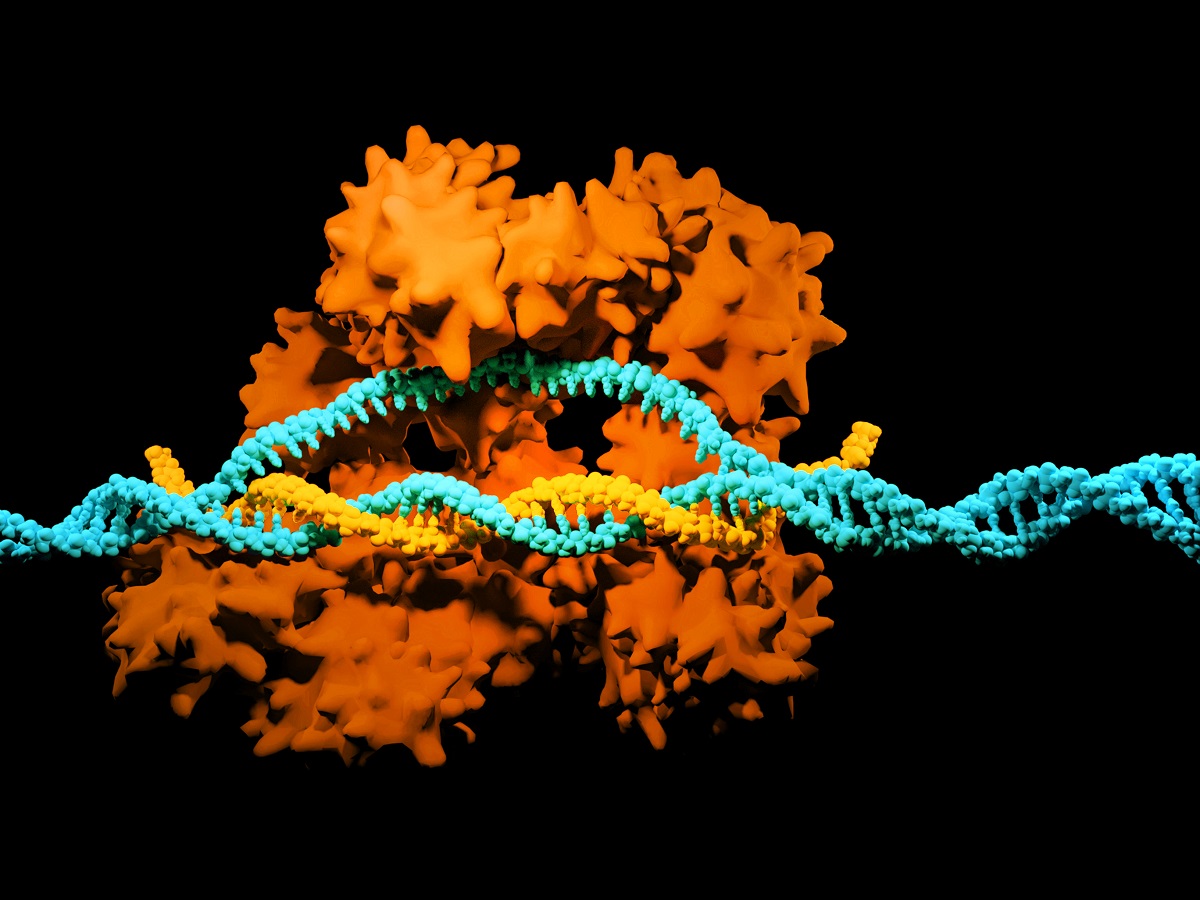
The Climate Change Committee (CCC) recently published (9 December 2020) a report detailing its recommendations for the Sixth Carbon Budget, which set out the steps the UK must take to achieve its goal of reaching net-zero greenhouse gas (GHG) emissions by 2050. One of the major recommendations from the report was the need to rapidly replace the use of primary fossil resources with low-carbon alternatives, such as biofuels.
Why biofuel?
The term "biofuel" can include any fuel derived from biological materials. This potentially includes a wide variety of things such as trees and plants, crops, algae, or agricultural or animal waste. Since biological feedstock material can be readily replenished, biofuels are generally considered to be a renewable source of energy.
Although the majority of biofuels have a lower energy content than conventional petroleum fuels, biofuels have the advantage of contributing approximately 60-90% less GHGs when compared with traditional fuel sources (total GHG emissions are dependent upon the specific feedstock material, location and bioconversion process). This is because energy crops take in CO2 as part of the photosynthesis cycle. Energy crops are therefore able to recycle the carbon dioxide that is released when the fuel product is burnt. In contrast, when fossil fuels are burnt they release carbon into the atmosphere that was removed from it by plants or animals millions of years ago.
Biofuels are not yet carbon-neutral due to the energy-needed to generate the feedstock (including, for example, fertiliser, machinery and processing). Nevertheless, bioconversion is one of the few large-scale technologies likely to be readily capable of achieving net-negative emissions. Biofuels can be used to generate energy directly by being used in power stations. They are also expected to play a pivotal role in helping to decarbonise the fuel supply, which is likely to be particularly important for heavy goods vehicles, ships and aircraft due to the difficulty associated with electrifying these modes of transport.
Biofuel classes
Biofuels are generally grouped into three main classes:
First-generation biofuels made from crop plants with high concentrations of carbohydrates (for example, potato, barley, wheat and corn). The main disadvantage of first-generation biofuels is that they require large areas of land, water and fertiliser to cultivate the feedstock material. As a result, first-generation feedstock tend to compete with food crops for cultivable land and water, which can lead to deforestation and also cause or exacerbate water shortages and lead to food scarcity by competing with food crops.
Second-generation biofuels made from non-food crops and feedstock that contain high amounts of lignocellulose. These crops tend to be grown on marginal land and require more limited amounts of water and fertiliser to grow. Although this has helped to alleviate many of the land-use issues cause by first-generation biofuels, the bioconversion of second-generation feedstock is notoriously difficult (and expensive) and there is still competition to develop a single efficient processing pathway. This has negatively affected funding as investors are reluctant to incur liability for high-cost processing centres, which risk becoming technically redundant.
Third-generation biofuels utilise tiny microorganisms such as algae and bacteria to produce biomass. Algae are photosynthetic organisms related to plants that grow in water and produce energy from carbon dioxide and sunlight. Algae can be used to produce hydrogen gas, bioethanol and biodiesel. The primary advantage of third generation biofuels is that they do not require high energy input and should not compete with arable land for the production of food. In contrast to conventional crops, micro-algae produce 15-300 times more oil for biodiesel production, making it a promising solution to the energy crisis¹. Algal products are expected to have a market value of more than $3.4 billion by 2025².
CRISPR to the rescue?
A limitation on third-generation biofuels is the efficacy of the microorganisms used, which has made it commercially challenging to produce biofuels on an industrial scale.
As is now increasingly well known, CRISPR-Cas9 is a naturally occurring prokaryotic defence system which has been reprogrammed to enable precise gene-editing. The Nobel Prize in Chemistry 2020 was awarded to Emmanuell Charpentier and Jennifer Doudna for its discovery. In summary, tailor-made guide RNA sequences are used to target nucleases to precise regions of the cell genome, where the nuclease is programmed to make an incision in the DNA. This triggers the cell's natural repair mechanisms. Scientists supply the cell with a DNA template to repair the gap, in principle allowing any target gene to be changed or replaced.
A number of groups are now using this gene-editing method to improve the organisms used in the bioconversion process, aiming to create highly-efficient phenotypes that are capable of producing biofuels on an industrial scale.
Genetic engineering to enhance lipid production is an increasingly viable option to improve the economics of biofuel production. Traditionally, groups focused on the manipulation of individual genes involved in the rate-limiting steps of the lipid biosynthesis pathway. This approach has achieved mixed success, mainly as a result of the multi-faceted nature of lipid biosynthesis regulation³.
Transcription factors
A more recent approach – made possible by the CRISPR-Cas9 system - has been to specifically target transcription factors which have been identified through high-throughput screening. Transcription factors are a promising option as they often target multiple regulatory points within the metabolic pathway⁴. While it has been shown that the up-regulation of key enzymes can increase lipid biosynthesis, a number of groups have been able to demonstrate substantial increases in lipid content through the development of knockout mutants.
This strategy focuses on blocking the metabolic pathways that are competitive to lipogenesis, for example, starch synthesis and lipid catabolism. A knock-out mutant of the phospholipase A2 gene, which is involved in the degradation of synthesised lipids, was shown to increase the total lipid content of microalgae by up to 64.25%⁵. Another notable example is the programme run by Exxon Mobile, which found that the knockdown of a single transcription regulator resulted in a twofold increase in lipid content⁶.
Carbon fixation is dependent upon multiple factors that operate through what is called the Calvin cycle. Key checkpoints in this cycle are the carboxylation of ribulose 1,5-biphosphate and its subsequent regeneration. Several enzymes assist in this process but ribulose-1,5-bisphosphate carboxylase/oxygenase (RubisCo) is the primary target for manipulation. Efforts to directly augment Rubisco have struggled as a result of the enzyme's complex kinetics. Therefore, groups have instead focused their efforts on factors which indirectly regulate Rubisco activity. Through manipulation of these factors, groups have been able to increase carbon fixation by maintaining a higher proportion of Rubisco with catalytically competent active sites.
Other uses
Another interesting possibility for third-generation biofuels is their potential to contribute to carbon capture storage systems. CO2 is a key resource for microalgae photosynthesis and carbon makes up approximately 35–65% of dry matter of microalgae biomass⁷. However, due to the relatively low concentration of CO2 found in the air, atmospheric CO2 is not effective for microalgae cultivation. It has therefore been proposed that the construction of a microalgae cultivation plant nearby a large emitter of CO2, such as a power station or relevant industry, offers a sensible alternative to help "store" CO2 emissions whilst increasing the photosynthesis efficiency of microalgae⁸.
Tracking innovation
Analysis of patent filing trends illustrates that interest in biofuels has been steadily growing since the mid-90's. This growth can be characterised by periods of intense activity, followed by downturns or periods of inertia⁹. Despite entering a brief period of decline following the emergence of shale gas and electric vehicles in the early-2010s, there are signs that we are at the beginning of a new wave of biofuel activity. Research by the International Energy Agency has shown that venture capital investment in bioenergy startups has increased eleven-fold since 2016. Research and development is expected to be further supplemented by governmental support, with a number of major economies stating or legislating specific biofuel deployment targets.
The US, China, Europe and Japan are the most heavily patented jurisdictions, although there is considerable divergence in regard to the technological subsections dominant in each jurisdiction. For example, the US has contributed to approximately 60% of the worldwide lignocellulosic patents¹⁰, while China is leading the way for patents related to the field of microalgae. Patent filings in the US and Europe are predominately made by industrial entities while the majority of patent filings made in Asia are by academic research institutions¹¹.
Most microalgae patents are related to specific strains and their cultivation under different conditions. Nevertheless, there a notable sub-sectors relating to up and downstream processes as well as methods to facilitate the extraction of microalgae products.
Osborne Clarke comment
Biofuels have long been proposed as the alternative fuel source of the future. Despite these high hopes, the commercialisation of biofuel technology to date has been hindered by high production costs, low yields and competition with food crops and other uses of land. These have limited the role biofuels have been able to play in decarbonising the economy. Despite many false starts, improvements in the identification, understanding and manipulation of key metabolic processes have now fuelled renewed optimism within the sector. It seems likely that companies will continue to focus on utilising tools such as CRISPR-Cas9 to create novel microorganism strains capable of producing biofuels on an economically viable scale.
As with a number of other renewable energy technologies, in at least the short term the success of biofuels is likely to depend on subsidies. As biofuels continue to increase in commercial importance, we can expect this to be reflected in the steps taken to protect them, with industry seeking to assert its dominance through the acquisition and enforcement of ever larger patent portfolios. This is already evident in more mature markets such as the United States and Europe, where corporations hold the majority of patent filings.
The UK government has announced that it expects to shortly issue a call for evidence to help inform the development of its biomass strategy, with a preliminary paper due to be published this summer. This will be followed by the publication of a new biomass strategy paper in 2022, which is intended to detail how biofuels can be best utilised to achieve the UK's net-zero GHG target by 2050.
Footnotes
- Muhammad Rizwan Javed et al., Current situation of biofuel production and its enhancement by CRISPR/Cas9-mediated genome engineering of microbial cells, Microbiological Research, Volume 219, 2019, Pages 1-11 | back
- Allied Market Research, Algae Products Market by Type, Source, Form, and Application: Global Opportunity Analysis and Industry Forecast 2018 – 2025, (2018) | back
- Sheehan, J et al., A look back at the US department of energy's aquatic species program: biodiesel from algae. National Renewable Energy Laboratory; (1998); 328, 1–294 | back
- Kumar G et al., Bioengineering of Microalgae: Recent Advances, Perspectives, and Regulatory Challenges for Industrial Application. Front Bioeng Biotechnol; (2020); 8:914 | back
- Shin YS et al., Targeted knockout of phospholipase A2 to increase lipid productivity in Chlamydomonas reinhardtii for biodiesel production. Bioresour Technol; (2019); 271:368-374 | back
- Ajjawi, I et al., Lipid production in Nannochloropsis gaditana is doubled by decreasing expression of a single transcriptional regulator. Nat Biotechnol; (2017); 35, 647–652 | back
- S. Chae, E et al., Hwang, H.S. Shin Single cell protein production of Euglena gracilis and carbon dioxide fixation in an innovative photo-bioreactor; Bioresour. Technol; (2006);  97 (2) pp. 322-329 | back
- Yoon Young Choi et al., Microalgae Bioenergy with Carbon Capture and Storage (BECCS): An emerging sustainable bioprocess for reduced CO2 emission and biofuel production, Bioresource Technology Reports; (2019); Volume 7, 100270 | back
- Li, Dongzi et al. A Quick Look Back at the Microalgal Biofuel Patents: Rise and Fall; Frontiers in bioengineering and biotechnology; (2020); vol. 8 1035 | back
- Hannes Toivanen et al., The emergence of patent races in lignocellulosic biofuels, 2002–2015; Renewable and Sustainable Energy Reviews, (2017); Volume 77, Pages 318-326 | back↵
- Li, Dongzi et al., A Quick Look Back at the Microalgal Biofuel Patents: Rise and Fall; Frontiers in bioengineering and biotechnology; (2020); vol. 8 1035 | back↵